By Wayne Gillam | UW ECE News
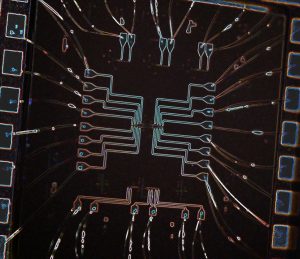
A UW research team led by UW ECE and Physics Associate Professor Arka Majumdar has moved quantum technology development a significant step ahead, demonstrating a new kind of silicon photonic chip that could work as a solid foundation for building a quantum simulator, one with useful applications in the real world. Shown above: An optical image of the electrically controlled coupled cavity array in the team’s silicon photonic chip. The image depicts the wiring structure and optical micrograph of the coupled cavity array. This visual, provided by Abhi Saxena, is an edge detected output that uses an optical microscope image as an input.
Today, we are living in the midst of a race to develop a quantum computer, one that could be used for practical applications. This device, built on the principles of quantum mechanics, holds the potential to perform computing tasks far beyond the capabilities of today’s fastest supercomputers. Quantum computers and other quantum-enabled technologies could foster significant advances in areas such as cybersecurity and molecular simulation, impacting and even revolutionizing fields such as online security, drug discovery and material fabrication.
An offshoot of this technological race is building what is known in scientific and engineering circles as a “quantum simulator” — a special type of quantum computer, constructed to solve one equation model for a specific purpose beyond the reach of a standard computer. For example, in medical research, a quantum simulator could theoretically be built to help scientists simulate a specific, complex molecular interaction for closer study, deepening scientific understanding and speeding up drug development.
But just like building a practical, usable quantum computer, constructing a useful quantum simulator has proven to be a daunting challenge. The idea was first proposed by mathematician Yuri Manin in 1980. Since then, researchers have attempted to employ trapped ions, cold atoms and superconducting qubits to build a quantum simulator capable of real-world applications, but to date, these methods are all still a work in progress. Recent advances in superconducting system design and fabrication have led to several successful implementations of prototypical quantum simulators that demonstrate small-scale quantum systems. However, there have been challenges in enlarging these systems to a usable size, as well as operating difficulties when attempting to use superconducting systems to simulate actual quantum materials.
Now, a UW research team led by UW ECE and Physics Associate Professor Arka Majumdar has moved this effort a significant step ahead, demonstrating in Nature Communications that a new kind of silicon photonic chip could work as a solid foundation for building a quantum simulator, one with useful applications in the real world. Majumdar is an expert in optics, photonics, and the development of quantum technologies. At the UW, in addition to his teaching and research responsibilities, he is a co-chair of QuantumX and a member of the Institute for Nano-Engineered Systems.
“We’ve shown that photonics is a leading contender for quantum simulation, and photonic chips are a reality,” Majumdar said. “We believe that these chips can play a very important role in building a quantum simulator.”
“This is a very good platform for realizing a useful quantum simulator that could be scaled to large sizes,” added Abhi Saxena, lead author of the paper and recent UW ECE alumnus. Saxena graduated in 2023 with his doctoral degree and now works for the National Institute of Standards and Technology (NIST) in Boulder, Colorado.
Other members of the research team include Arnab Manna, a doctoral student in the physics department and UW ECE Assistant Professor Rahul Trivedi, a quantum systems expert who assisted the group with theoretical aspects of their research.
The advantages of a silicon photonic chip — scalable, measurable, programmable
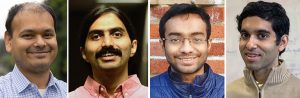
The UW research team. From left to right, UW ECE and Physics Associate Professor Arka Majumdar, lead author and recent UW ECE alumnus Abhi Saxena (Ph.D. ‘23), physics doctoral student Arnab Manna, UW ECE Assistant Professor Rahul Trivedi
Photonics is a branch of optics (the study of behavior and properties of light) that applies generation, detection, and manipulation of light to enable a wide range of technologies, such as lasers, fiber optics, and light-emitting diodes (LEDs). A key advantage photonics has over other methods of building a quantum simulator platform is that photonic devices can be fabricated in CMOS foundries, which have been used for decades to produce semiconductor chips.
“The fabrication process that we have for this chip can directly latch onto the already well-matured silicon fabrication that we do for transistors and other computer chips,” Saxena said. “Whereas for other quantum simulator platforms that’s not feasible, even though many of them have already demonstrated prototypical devices.”
As a case in point, the research team created their silicon photonic chip at the Washington Nanofabrication Facility on the UW campus. Their fabrication method will help lower production costs for building a quantum simulator, and perhaps more importantly, make it possible to scale the chip up enough for it to be usable in a wide range of quantum simulation devices.
At the heart of the chip the team designed is a “photonic coupled cavity array.” This array is a pseudo-atomic lattice made up of eight photonic resonators. It is a place where photons can be confined, raised and lowered in energy, and moved around in a controlled manner, essentially forming circuits. Important technical innovations by the team related to the array include creating a mathematical algorithm that allowed them to map, or characterize, the chip in detail, using only information available on the boundaries of the chip, and designing a new kind of architecture for heating and independently controlling each cavity in the array, which let the team program the device. According to Majumdar and Saxena, these two innovations on a silicon photonic chip have never been accomplished before.
“We are demonstrating everything on a chip, and we have shown scalability, measurability and programmability — solving three of the four major obstacles to using a silicon photonic chip as a platform for a quantum simulator,” Majumdar said. “Our solution is a small size, it is not misalignment-prone, and we can program it.”
What the future holds
Moving forward, the research team seeks to solve what they see as the fourth, and final, major obstacle to building a fully realized quantum simulator, creating a condition called “nonlinearity.” Unlike the electrons commonly found in electronic circuits, which repel each other because of their negative electrical charge, photons, by their nature, do not interact with each other. An equivalent interaction is needed in a quantum simulator to create nonlinearity and complete the circuitry. The team is currently exploring several different approaches to address this issue.
Also on the research team’s agenda is to fine-tune their silicon photonic chip, optimizing it for standard chip foundries, so the chip can be manufactured at semiconductor fabrication plants around the world. Majumdar and Saxena both said that this aspect of development would be, relatively speaking, an easier hurdle, and they expressed optimism about the impact their chip will have.
“Through this work, we presented a solid foundation for a platform that demonstrates photonics and the semiconductor-based technology we are using as viable alternatives to create quantum simulators,” Saxena said. “I think that up until now, many in the scientific and engineering communities have generally avoided considering photonics for this purpose. But our work shows that it is realistically possible, so it is a very good incentive for more people to begin moving in this direction.”
For more information about the research described in this article, read the team’s paper in Nature Communications or contact Arka Majumdar.