Article by Andrew Engleson | UW Magazine
Quantum physics is weird. Many an undergrad has been baffled by Schrödinger’s cat in a box which could be both dead and alive until the box is opened. Some of us ponder how light exists as both a wave and particle. And our pandemic quarantine might give us time to work on understanding the notion of action at a distance in which two entangled particles, separated by a great distance, change state instantaneously if one is observed.
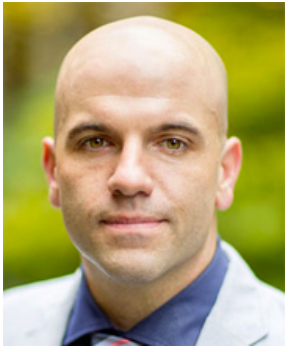
Jim Pfaendtner
It turns out these and other bizarre components of quantum physics are the foundation for a new kind of computer, one that promises to be substantially faster and more powerful than any that exists today. And UW researchers in physics, computer science, chemistry, engineering and materials science are training leaders in the burgeoning field of quantum information science and technology, or QIST.
QIST offers radically new advances in a variety of fields as well: ultrasensitive sensors to one day measure the firing of individual neurons in the brain, or completely secured encrypted communication.
Jim Pfaendtner, chemical engineering professor and chair of UW’s Chemical Engineering Department, notes that quantum computing could force us to jettison Moore’s Law, the dependable rule of thumb that asserts computing power tends to double every two years.
“You’ll have a radical change in the type of a certain class of calculations—the scaling is massively higher,” Pfaendtner says. “So the number, the extent of calculations that you can begin to conceive of doing will really change overnight if this technology comes to pass.”
“Today’s crypto-keys will not be secure when quantum computing is realized. Because the computers will be exponentially faster.” – JIM PFAENDTNER, UW CHEMICAL ENGINEERING PROFESSOR
Calculations that would take thousands of years on classical computers could conceivably take just a few hours. The benefits are many, but there’s one striking potential impact: current security and encryption would be obsolete. “Today’s crypto-keys will not be secure when quantum computing is realized,” Pfaendtner says. “Because the computers will be exponentially faster.”
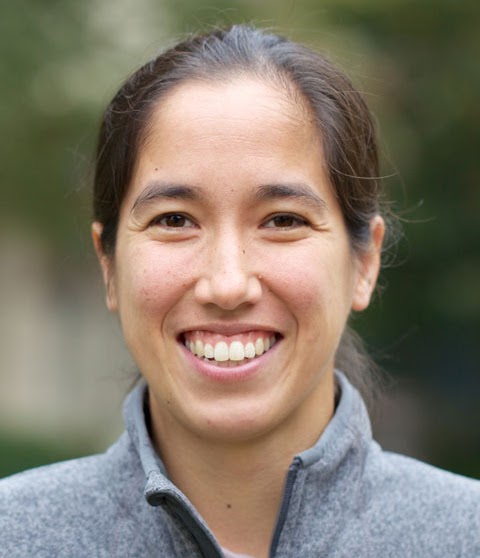
Kai-Mei Fu
Not surprisingly, the U.S. government has taken notice, dedicating more than a billion dollars in 2020 to research efforts. In the past four years, UW has received $30 million in funding for QIST research, says Kai-Mei Fu, associate professor of physics and electrical and computer engineering as well as a researcher with the Pacific Northwest National Laboratory (PNNL).
Fu helps lead a lineup of regional quantum collaborations including Northwest Quantum Nexus, a research partnership among UW, Microsoft and PNNL. She’s also a leader of the Quantum X initiative, which brings together UW researchers across disciplines. “Quantum X is a very typical bottom-up University of Washington endeavor,” Fu says. “We realized there are a lot of people doing quantum on campus. Our main goal is to connect everyone.”
Quantum X brings together principal investigators at UW in materials science, physics, electrical and computer engineering, and other disciplines integral to creating a quantum computer. “Building up connections between these disparate groups of people is not easy,” says Nathan Wiebe, a senior scientist at PNNL and until recently a UW affiliate associate professor of physics. “I think the hardest part about building a quantum computer is going to be trying to figure out how to get everybody able to talk to each other. We all need to be involved to get this to work.”
A fascination with diamonds
What makes a quantum computer different from a standard computer is the qubit. A classical computer works using bits, which represent information as a string of values of either 0 or 1. Qubits store information in a single atom or particle. But rather than using a solid value of 0 or 1, the qubit stores a range of possibilities. Wiebe explains it as the difference between looking at which side of a coin is face up on a table (a bit of either heads or tails), versus a flipped coin that’s covered by your hand; you know the probability of it, but don’t actually know for certain if it’s heads or tails.
Pfaendtner likes the analogy of a 3-D maze to describe qubits. “Every time you come to a junction, classically, you’d pick one direction and go until you reach the dead end. You’d keep a map of that in your mind, or your memory. Then you would go back when you reached a dead end. You’re never going to guess the maze the first time correctly, but you will eventually solve the maze.
“In a quantum computer, in a qubit, instead of picking one direction, you pick both directions. So you simultaneously explore both paths. Every time you come to a junction there is the ability to not have one state, but have multiple states. This is the fundamental paradox of quantum physics that’s difficult for everybody to understand.”
The power of qubits comes from their ability to add these probabilistic wave-functions of information together, creating an exponentially more powerful and much faster way to do calculations.
But it turns out that creating a working qubit is fiendishly difficult. You need to manipulate a single atom or particle, which isn’t easy. Atoms interfere with one another, making precise measurements difficult unless you can isolate them. “We want to build a big, powerful, thick box to secure our quantum information,” Wiebe says. “But we don’t want it to be so secure that we can’t read it.”
That why Kai-Mei Fu is fascinated with diamonds.
“Part of the allure of a diamond isn’t that it’s a beautiful material,” she says. “It has nice properties, has very extreme properties. Part of it is more mundane—it’s pure enough that I can work with it without interference from a noisy environment.”
“What’s really key is we’re bringing together students from different departments.” – KAI-MEI FU, PHYSICS AND ELECTRICAL & COMPUTER ENGINEERING PROFESSOR
Fu and her colleagues specialize in creating minuscule defects in otherwise perfectly pure diamonds to manufacture qubits. Inside the lattice of carbon atoms that make up a diamond, you can sneak in two nitrogen atoms. This creates tiny flaws, or “vacancy centers,” that can, when brought down to super-cold temperatures, be manipulated to store information.
The trick is integrating those tiny empty spaces into an actual circuit. Much ballyhoo surrounded the Google announcement in 2019 that it had built a rudimentary 53-qubit quantum computer that achieved “supremacy”—quickly solving a problem that classical computers would take much longer to figure out. Then last year, IBM announced it had constructed its own 64-qubit processor. But the results of these efforts are still tenuous, and just how successful these first efforts have been is hotly debated among scientists.
One big problem with qubits is their relatively high error rate. Even after the atoms are isolated and manipulated, one concern is decoherence—a quantum effect that’s essentially a random change in the atom’s state, which can be caused by an electric or magnetic field, stray radiation or other environmental factors.
What Fu and her UW colleagues have focused on is creating improved interfaces between those tiny defects and a larger circuit that can manipulate the information contained in them. Working with UW’s Nanofabrication Facility, Fu says, “We can make devices that couple these defects to these photons. That’s huge.”
Three of Fu’s colleagues in the Department of Electrical & Computer Engineering (UW ECE), Mo Li, Arka Majumdar and Karl Böhringer, received a National Science Foundation (NSF) grant last fall to work on developing a microchip-sized steering system that coordinates multiple laser beams—which could eventually link more than 1,000 qubits. “It’s a huge engineering challenge controlling all these beams,” Fu says.
In another multidiscipline effort, Fu is leading a $3 million traineeship program also funded by the NSF that brings together UW graduate students across different fields to collaborate on QIST research. Fu says, “What’s really key is we’re bringing together students from different departments.”
The architecture of a revolution
For Martin Savage, a professor of physics at the UW’s Institute for Nuclear Theory, one missing puzzle piece is imagining how to actually use quantum computers. “One of the things that we need to understand especially is how to use a quantum computer to solve problems,” says Savage. “We kind of don’t know how do that at the moment.”
Using existing supercomputers or even just standard laptops, Savage and his colleagues are trying to simulate how quantum computers might be applied to unsolved problems in fundamental physics. He and UW colleagues Silas Beane and David Kaplan have created the InQubator for Quantum Simulation (IQuS), which is beginning the work of figuring out which research questions quantum computers would be best applied.
Imagining those uses can sometimes expose current limitations. Fu notes this in her work with diamonds. “To give you a scope of the problem, even though we’ve removed one atom from a crystal, actually simulating how that crystal should behave is hard. That’s a quantum mechanical problem, one that you practically need a quantum computer to do.”
Wiebe estimates that it may be as long as 20 years before a truly functional quantum computer is operational. And that’s even allowing for the rapid pace the technology has advanced at in the past 10 years.
Wiebe sums up the challenge this way. To do useful calculations, a million-qubit chip would be required. With existing technology, he says, we “would need at present to make a chip that’s about 1-meter square and stored at like 10 to 30 millikelvin [near absolute zero]. The control electronics would take up several football fields and cost over a billion dollars.”
A regional hub for quantum research
Just what quantum computers will be applied to is a fascinating and potentially controversial question.
Wiebe notes one surprising application: fertilizer production. The chemical process for creating ammonia-based fertilizer has been around for over a hundred years. It’s fairly simple process, but one that consumes close to 1% of the world’s total energy use.
But now we know that bacteria have evolved to make ammonia at room temperature using an enzyme called nitrogenase. Using that enzyme on a large scale could significantly reduce global energy consumption. But the process isn’t well understood and can’t be replicated beyond a single cell. “Despite 100 years of trying,” Wiebe says, “nobody has actually been able to crack the problem of how exactly this kind of molecular knife that bacteria have discovered actually works.”
The complex chemistry—which includes heavy metals such as iron and molybdenum—can’t be modeled using existing computers. It would potentially take thousands to millions of years. But with a fully functional quantum computer, Wiebe predicts “we could actually simulate it in the span of a few hours.”
Savage points to another application on a much larger scale. “Take for instance, colliding neutron stars,” he says. “What happens in the densest part of that? Using a classical computer, we still don’t have answers with the precision we need.”
The potential to create a computer that can bypass existing cryptographic encryption is driving governments in the U.S. and China to massively scale up QIST funding. Wiebe says having a strategy now will help mitigate future security risks.
“Twenty years is enough time for us to develop some good tools. We really need to build up and make sure these things are reliable and can hold up against ordinary hackers in addition to the quantum hackers we’re going to be worried about in 20-plus years.”
Strangely enough, QIST also allows for the creation of perfectly secure communication networks. Based on quantum principles such as entanglement and the impossibility of copying a quantum state, quantum keys are packets of information that always bear a trace if observed.
“What makes [quantum keys] completely secure is that as soon as someone tries to copy, disturb, or see the message, it leaves an imprint on the message that’s detectable,” says Fu. Even a quantum computer wouldn’t help overcome this perfectly secure key.
At the moment, the implications are merely theoretical. But as QIST researchers like those at UW advance and refine the technology, hard decisions will have to be made about who can use these tools. “We have to decide when we want to use this,” says Fu, “and when do we not want to use this?”
For now, the researchers are focused on advancing the technology, bolstered by a vibrant quantum research community in the Pacific Northwest. The UW, Microsoft, Amazon, and Intel, as well as PNNL and a host of quantum startups such as D-Wave Systems and 1QBit (both in British Columbia) are all making Cascadia a magnet for QIST research.
“One of the things that really attracted me to UW and the Pacific Northwest for quantum is the amazing synergies that are possible between all of these different organizations,” says Wiebe. “We’ve got an amazingly strong computer science department at UW. We’ve got very strong chemistry, as well as electrical engineering and physics departments—and surrounded by a wonderful collection of industrial partners.”
In a decade or two, we’ll know if computers are ready to take the next quantum leap.