By Anna Wirth-Singh | UW Department of Physics
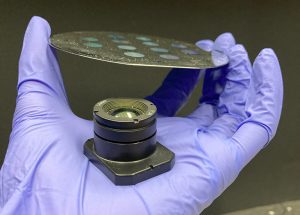
Ultra-thin meta-optics have the potential to make imaging systems lighter and thinner than ever. Using a new inverse design framework, a UW ECE-led research team has demonstrated broadband thermal imaging with meta-optics for applications ranging from consumer electronics to thermal sensing and night vision. Shown above, a side view of a fabricated wafer containing meta-optics held above a conventional refractive lens. The meta-optics were developed by a multi-institutional research team led by UW ECE and Physics Associate Professor Arka Majumdar. Photo by Anna Wirth-Singh.
Long-wavelength infrared (LWIR) imaging holds critical significance across many applications, from consumer electronics to defense and national security. It finds applications in night vision, remote sensing, and long-range imaging. However, the conventional refractive lenses employed in these imaging systems are bulky and heavy, which is undesirable for almost all applications. Compounding this issue is the fact that many LWIR refractive lenses are crafted from expensive and limited-supply materials, such as germanium.
The next generation of optical systems demands lenses that are not only lighter and thinner than ever before, but also uphold uncompromising image quality. This demand has fueled a surge of efforts to develop ultra-thin sub-wavelength diffractive optics, known as meta-optics. Meta-optics, in their simplest form, consist of arrays of sub-wavelength scale nanopillars on a flat surface, with each pillar introducing a local phase shift to light passing through. By strategically arranging these pillars, the light can be controlled to produce steering and lensing. While conventional refractive lenses are close to a centimeter thick, meta-optics are about 500 microns thick, which dramatically reduces the overall thickness of the optics.
However, one challenge with meta-optics is strong chromatic aberrations. That is, light of different wavelengths interacts with the structure in different ways, and the result is typically a lens that cannot simultaneously focus light of different wavelengths in the same focal plane. Largely because of this issue, meta-optics have not yet fully replaced their refractive counterparts despite the benefits in size and weight reduction. In particular, the area of LWIR meta-optics is relatively unexplored compared to visible wavelength meta-optics, and the potential advantages of meta-optics over conventional refractive lenses are significant given the unique and extensive applications of this wavelength range.
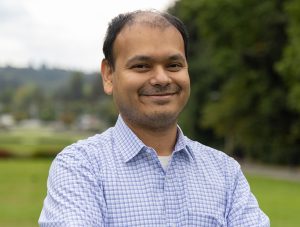
UW ECE and Physics Associate Professor Arka Majumdar. Photo by Ryan Hoover.
Now, in a new paper published in Nature Communications, a multi-institutional team of researchers, led by UW ECE and Physics Associate Professor Arka Majumdar, has introduced a new design framework termed “MTF-engineering.” The modulation transfer function, or MTF, describes how well a lens maintains image contrast as a function of spatial frequency. This framework addresses the challenges associated with broadband meta-optics to design and experimentally demonstrate thermal imaging with meta-optics in laboratory and real-world settings. The team built upon already successful inverse design techniques by developing a framework which optimizes both the pillar shape and the global arrangement simultaneously. UW ECE-affiliated team members included recent alumni Luocheng Huang (the paper’s lead author) and Zheyi Han, postdoctoral researchers Saswata Mukherjee, Johannes Fröch, and Quentin Tanguy as well as UW ECE Professor Karl Böhringer, who is the director of the Institute for Nano-Engineered Systems at the UW.
Leveraging artificial intelligence and a new inverse design framework
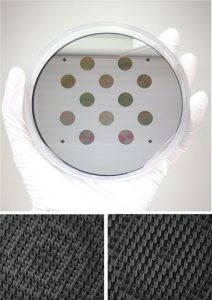
Meta-optics, in their simplest form, consist of arrays of sub-wavelength scale pillars on a flat surface, with each pillar introducing a local phase shift to light passing through. By strategically arranging these pillars, the light can be controlled to produce steering and lensing. (Above) A full view of a fabricated wafer containing meta-optics. (Below) Scanning electron microscope images of the nanopillars contained within the team’s meta-optics. These meta-optics contain both complex light scatterers (left) and simple scatterers (right). Photos provided by Anna Wirth-Singh.
One key innovation in the research team’s approach is the use of artificial intelligence — a deep neural network (DNN) model — to map between pillar shape and phase. In an inverse design process for large area optics, it is not computationally feasible to simulate how the light interacts with each pillar at each iteration. To solve this problem, the authors simulated a large library of nanopillars (also called “meta-atoms”) and used the simulated data to train a DNN. The DNN enabled a quick mapping between scatterer and phase in the optimization loop, allowing the inverse design of large-area optics containing millions of micron-scale pillars.
Another key innovation in this work is the figure of merit (FoM), leading to the framework being termed “MTF-engineering.” In inverse design, one defines an FoM and computationally optimizes the structure or arrangement to maximize the FoM. However, it is often not intuitive why the produced result is optimal. For this work, the authors leveraged their expertise in meta-optics to define an FoM that is intuitive.
Majumdar explained, “The figure of merit is related to the area under the MTF curve. The idea here is to pass as much information as possible through the lens, which is captured in the MTF. Then, combined with a light computational backend, we can achieve a high-quality image.” He continued, “The figure of merit reflects what we intuitively know about optics. This particular FoM is optimized when all the wavelengths perform equally well, thus constraining our optics to have uniform performance over the specified wavelengths without explicitly defining uniformity as an optimization criterion.”
This approach, combining intuition from meta-optics and a light computational backend, significantly improves performance compared to simple metalenses.
The authors fabricated their designed optics from a single silicon wafer, which is promising for future applications involving germanium-free LWIR imaging systems. While acknowledging that there is still room for improvement to achieve imaging quality comparable to commercial refractive lens systems, this work represents a significant step toward that goal.
The researchers have generously made their MTF-engineering framework, named “metabox,” available online via GitHub, inviting others to use it for designing their own meta-optics. The research team expressed excitement about the potential works that may emerge from the utilization of metabox in the broader scientific community.
This article is an adaptation of a blog post available at Springer Nature Research Communities. For more information about the research described above read, “Broadband thermal imaging using meta-optics” in Nature Communications or contact UW ECE and Physics Associate Professor Arka Majumdar.